Carbon Nanotubes (CNT) and graphene are the flagship of bottom-up manufacturing, offering high strength and transport properties which make them suitable for multi-functional composites. My group addresses the challenges and exploits the opportunities imparted by self-organizing architectures of carbon nanomaterials.
To advance graphene manufacturing, we are leading a community effort to crowd-source graphene synthesis recipes and automated image analysis into an open database to enable machine learning-driven growth of nanomaterials. (Collaboration: Elif Ertekin)
The lamination of 2D materials on crystalline substrates leads to emerging electronic and mechanical properties such as periodic faceting, surface stiffening, and toughening. We study the interfacial mechanics of graphene-metal and graphene-polymer composites to control the atomic mechanisms of strengthening in these nanocomposites.
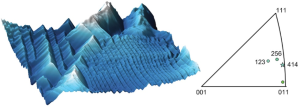
When graphene is synthesized on the surface of a metal catalyst, interesting facets form. We develop methods to study the crystallography and interfacial mechanics of these facets. This phenomena affects the transport of graphene, and its suitability for device integration.
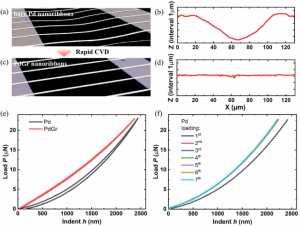
When graphene is synthesized on this metal ribbon, they become stiffer and tougher. We studythis mechanical behavior using a strip indentation approach where the material is almost intension due to the small thickness and long suspended spans
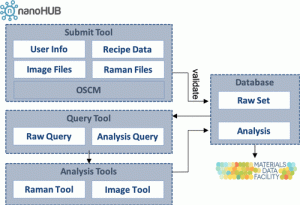
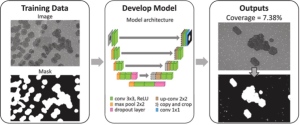
We are interested in using crowd-sourced data to optimize graphene synthesis conditions to obtain high quality graphene at economical cost and large scales. We are developing software tools to enable the scientific community to share data and machine learning tool to use this data to advance synthesis.
Hierarchical fiber geometries are responsible for the powerful contraction of natural muscles and tendons. However, only qualitative understanding exists today for the relation between the helical morphologies and the muscles’ performance. We work on synthetic actuators, known as coiled artificial muscles, which rely on highly twisted and coiled polymeric fibers to create large contractions when stimulated. The stimulation can be via thermal, electrothermal, chemical, or electrochemical expansion. We develop theoretical modeling, novel architectures, and design rules for artificial muscles.
We use artificial muscles in powering insect-scale jumping robots. We demonstrated system integration of coiled artificial muscles in battery-operated small-scale robotics where they offer large strokes in compact volumes.
At small scales, extreme flexibility magnifies the effects of capillary forces, which eventually dominate over the elastic restoring forces. My group performed basic experiments to unravel the physics of hydrodynamic elastocapillarity to architect fibers and fins. We discovered fiber self-organization phenomena emerging due the interplay of capillarity and hydrodynamic fluid flow. We recently demonstrated a new concept of polymorphic texture integrated systems (Polytexis) where morphing fins control the texture of a surface by integrated microfluidic network to direct the hydrodynamic and capillary driven motion of these fins.
Nonlinear architected materials enable unusual energy absorbing as well as elastic and acoustic energy propagation. We use additive manufacturing (AM) to produce architected materials which, owing to internal buckling, realize ultra-high energy absorption and an ultrawide flat plateau. We use machine learning to optimize their geometry and develop new smart metrology algorithms to relate their geometry to their mechanical behavior.
In parallel with the microscale studies described above, we advanced emerging nonlinear dynamic concepts using macroscale desktop demonstrations, precisely engineered with metal flexures to conserve elastic energy like “Swiss clocks”. We have recorded the first experimental demonstration of the acoustic analog of the quantum Landau Zener Tunneling (LZT) effect. In LZT, acoustic waves travel in a nonlinear waveguide made from geometrically tailored flexures through the propagation of breathers – nonlinear wave packets – only when the wave energy is over a certain barrier. On the microscale, we study the acoustic wave propagation in arrays of coupled drumhead resonators, which undergo buckling-induced phase transition leading to loss of transmission.
Our group develops new additive manufacturing processes using polymeric materials and composites. We are interested in self-curing polymers, those which undergo frontal ring opening metathesis polymerization, and hence use low energy as their polymerization reaction is self-energized. The resulting material is a high-performance thermosetting polymer with high stiffness, strength, toughness, and thermal stability. We are also interested in developing methods to upcycle these polymers.
Our group also developed new 3D printing processes for polymeric materials at small scales, much smaller and much faster than the existing state-of-the-art, to make geometrically functional, bio-inspired microparticles.